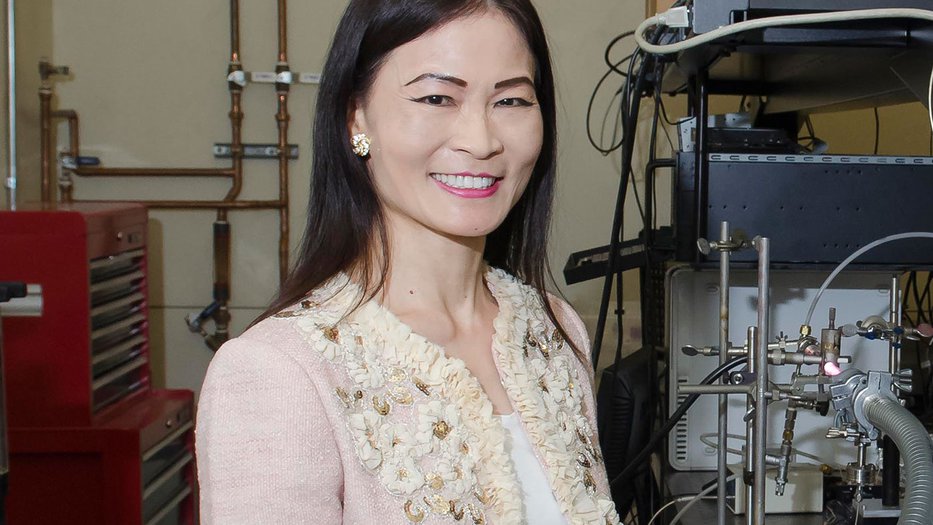
Ask a Caltech Expert: Nai-Chang Yeh on Quantum Materials
How Will Quantum Science and Technology Change Materials, and Vice Versa?
We asked Professor Nai-Chang Yeh, an experimental physicist and former Fletcher Jones Foundation co-director of Caltech's Kavli Nanoscience Institute, to answer this question. She measures, creates, and engineers materials at the nanoscale to investigate and harness their quantum electronic and optical behaviors.
The future of technology hinges on quantum materials. New materials have the power to advance conventional technologies, and they are also required for quantum computing qubits as well as other quantum technologies such as quantum memories, quantum teleportation, and quantum networks.
Even if we are just talking about conventional technologies, we want them to have a lot of capabilities for computing, sensing, and memory storage. And when we try to increase those capabilities, we have to work at the nanoscale. At these tiny scales, the physics concepts can be totally different from what they are at large scales.
When you go down to the nanoscale, you can never avoid quantum. Things like quantum entanglement and quantum fluctuations become very important. These days, industrial companies come to physicists for help. You need to know quantum science to solve frontier technological problems, make improvements, and come up with new ideas.
Electronics are hitting a bottleneck as technologies go down to these tiny scales. Just think: In an iPhone, there are many billions of transistors. Silicon, the conventional material for transistors, is reaching its limit because the demands for extreme device densities lead to formidable power consumption and serious materials issues at the nanoscale. The applications for nanoelectronics are everywhere, from cell phones and computers to the internet of things, as well as new technologies to harvest, store, and transmit information—essentially all those smart things that we connect online.
Therefore, new quantum materials are needed to further advance nanoelectronics in addition to quantum technology. For instance, we can make a lot of improvements in nanoelectronics by combining silicon with graphene, which consists of a single atomic layer of carbon atoms forming a honeycomb lattice.
What is a quantum material? To me, everything is quantum—when you consider materials at the scale of atoms, they are held together because of the overlaps of electronic wave functions from the constituent atoms, which is clearly a quantum mechanical phenomenon. When we try to describe the electronic structures of materials, we have to use concepts from quantum mechanics and cannot rely on classical approximations. Initially, physicists cooked up the phrase "quantum materials" just to refer to strongly correlated materials, and we later added topological and engineered materials.
In strongly correlated materials, the electronic properties are interdependent. Unlike simpler materials such as noble metals or typical semiconductors, when you study strongly correlated materials, you cannot make problems simpler by looking at one property and just approximating the rest. The electrons' charges, spins, and orbits, as well as the background ionic motions (known as "phonons"), affect each other.
Topological materials are materials whose properties are invariant under smooth deformations. These materials can exhibit interesting electronic states on their surfaces that reflect what is happening farther inside the bulk.
Today, researchers can construct engineered quantum materials with desired properties using laser light, growing methods, or nanofabrication. With lasers, we can trap, chill, and arrange tens or hundreds of atoms or molecules. We can also grow materials one atomic layer at a time. And we can use nanofabrication instruments to build new materials, control their electronic, optical, magnetic, and even superconducting properties, and sense how they behave over time. Often, we combine methods. For example, in my group, we grow graphene and then lay it on a substrate with nanostructures fabricated by nanotechnology, which stretches the monolayer graphene at the peaks of the nanostructures to make periodic graphene "wrinkles" with unique electronic properties.
Now that we can make nanoscale materials, people may think that we can just choose the properties that we want, then make the materials. But that's often hard. There are so many elements in the periodic table; what is the right combination? Which structures and construction methods are best? It's an ocean of possibilities. I hope that with machine learning, computers will come up with better recommendations than human minds can.
Frontier technologies are intertwined with quantum science. If we want technology to progress steadily, the development of quantum materials is extremely important. I think we can do it. When engineers from industry come with questions, I see that the issues they encounter are actually wonderful scientific problems.
— Nai-Chang Yeh, Professor of Physics
You can submit your own questions to the Caltech Science Exchange.