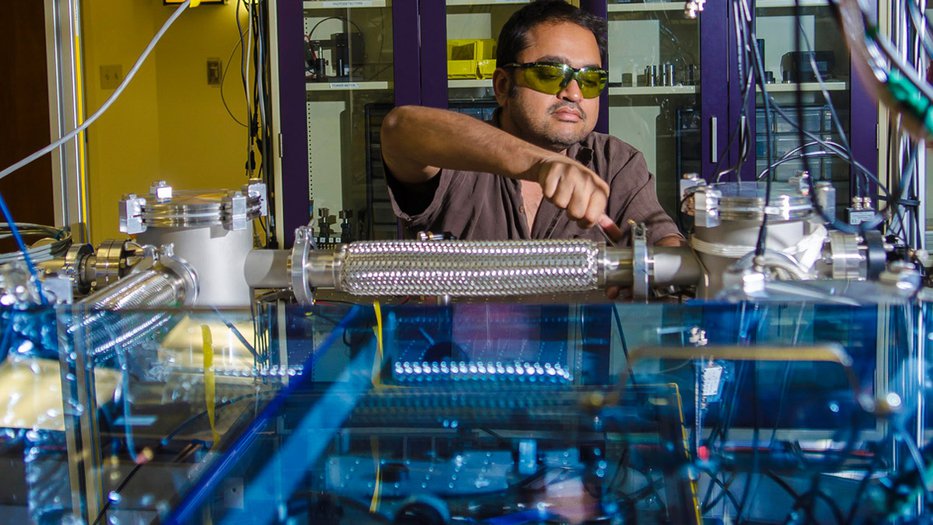
Ask a Caltech Expert: Rana Adhikari on Quantum Sensors
How Will Quantum Sensors Change Gravitational-wave Detection and Other Fields?
We put this question to professor Rana X Adhikari, who studies fundamental physics, in part, by designing the optical systems and sensors that LIGO, the Laser Interferometer Gravitational-wave Observatory, uses to detect and measure gravitational waves.
Gravitational waves ripple out from collisions and explosions in space and reach Earth as extremely tiny vibrations in spacetime. For decades after Albert Einstein predicted their existence, these waves were thought to be too weak to detect, even if they did exist.
Then the founders of LIGO decided to try to develop detectors that could measure these spacetime distortions from as far away as hundreds of millions of light years. The way those physicists thought was: If we want to measure a little thing that's shaking, what are all the rules of the universe that allow us to do it, and what gets in the way? That is the right kind of thinking.
That led to LIGO's first success, in 2015, and the many black hole mergers it has detected over the last five years.
Today, even after we've improved its sensing capability using squeezed light, the LIGO technology is at the limit of what can be done using conventional techniques. We want to develop another idea that uses basic physics to advance astronomy. We need a huge sensitivity improvement to go from detecting black hole mergers to detecting quieter phenomena, even gravitational waves from the beginning of the universe.
As it turns out, the same tools that people use to figure out how to do quantum computing without a lot of errors can be used to make quantum measurements better. In the last five years, everything I have been reading comes from the quantum information and computing community.
We want to have devices that utilize billions of entangled things, photons of light in this case, working in lockstep. Then we could set up correlations in our sensors that concentrate quantum uncertainties in pulses so that at alternate times, the uncertainty is close to zero and we can measure as precisely as we want.
But whenever you hear about quantum, it's delicate, right? Don't touch it. Don't talk about it. Don't look at it. Or else it won't be quantum anymore. That's no good! We want it to be super robust.
The trick of getting quantum mechanics to help in sensing is that we have to make a system that doesn't interact with the rest of the world. A dust particle or the magnetic field from a phone could pollute it with noisy, dirty molecules, and our system starts acting less quantum (more classical) and loses all the fanciness that we've worked so hard to engineer.
If you look at DNA, it has self-repair capabilities. We want to do the same kind of thing with quantum technologies. We'd like our sensor to know what it is supposed to be doing, detect if it has been disturbed by the environment, and constantly clean itself. There's a quote attributed to Archimedes along the lines of, "Give me a big enough lever, and I can move the world." My version would be, "Give me some quantum-measurement-excited grad students, and I can measure the universe."
The great challenge of detecting faint gravitational waves is just one scientific prize we can imagine as sensors improve by many orders of magnitude. Just as we've all become accustomed to having transistors in all of our electronic devices, we should imagine that we would have quantum-enhanced circuitry in all of our modern devices in a couple of decades.
Of course, when physicists bring up how we can use quantum mechanical devices for practical things, skepticism is well founded. We usually don't know what's involved on the engineering side or in the other sciences. But when we work with people who do, there's a lot that we can do together.
For example, one of our collaborators at UC Berkeley, the physicist Holger Müller, wants to help biologists image viruses using X-rays. But if you shoot X-rays at regular lenses, they get burnt and destroyed. So he took our approach, in which we make high-power laser cavities, and used those laser beams as lenses for X-rays. Using this technique, he was able to get high-resolution biological images. It worked because he collaborated with biologists, learned what they really need, and worked toward that using physics.
These advances happen. It just takes the kind of people who say: Let's sit down and talk about what you need and how we can improve things. What can I do in the whole chain of events that is needed to make it work?
—Rana X Adhikari, Professor of Physics
You can submit your own questions to the Caltech Science Exchange.