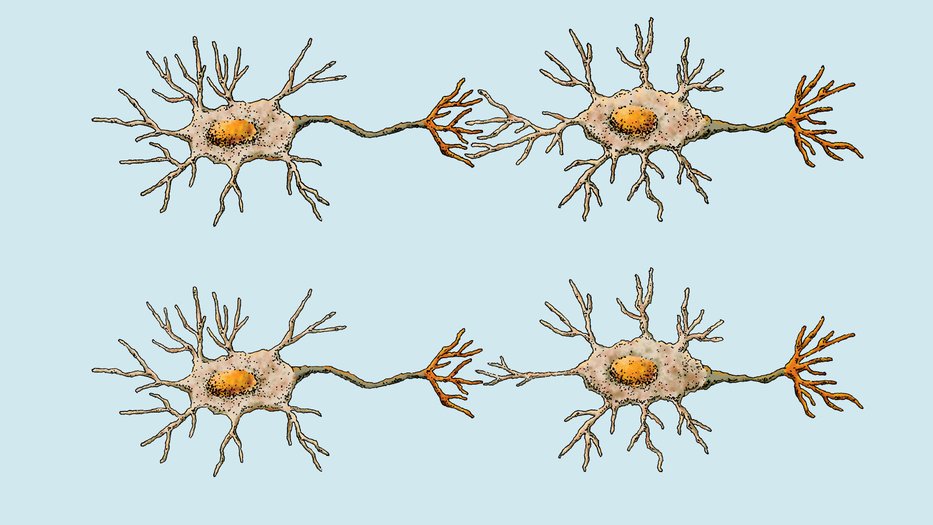
How Do Neurons Work and Change Over Time?
Neurons are the primary cells responsible for receiving and sending signals throughout the brain and nervous system. Like a person in a crowded room, each neuron can interact with others nearby, letting it direct and modify signals locally. Local signals can get passed on within groups of cells, permitting a signal to travel longer distances. Some neurons, called projection neurons, communicate with more distant parts of the brain or directly with muscles in our body. The human brain contains approximately 86 billion neurons, with trillions of connections among them.
Neurons
A neuron has three parts: the cell body, dendrites, and the axon (Figure 1). The cell body contains the small functional structures called organelles, which are necessary for the cell to survive. Dendrites extend outward from the cell body like many microscopic branches and are responsible for receiving most signals from other neurons. If a strong signal is received by a dendrite, it travels through the cell body and along the axon, an extended part of the cell. At the end of the axon, the signal gets passed on to the next neuron across a synapse, the small specialized structures through which neurons communicate.
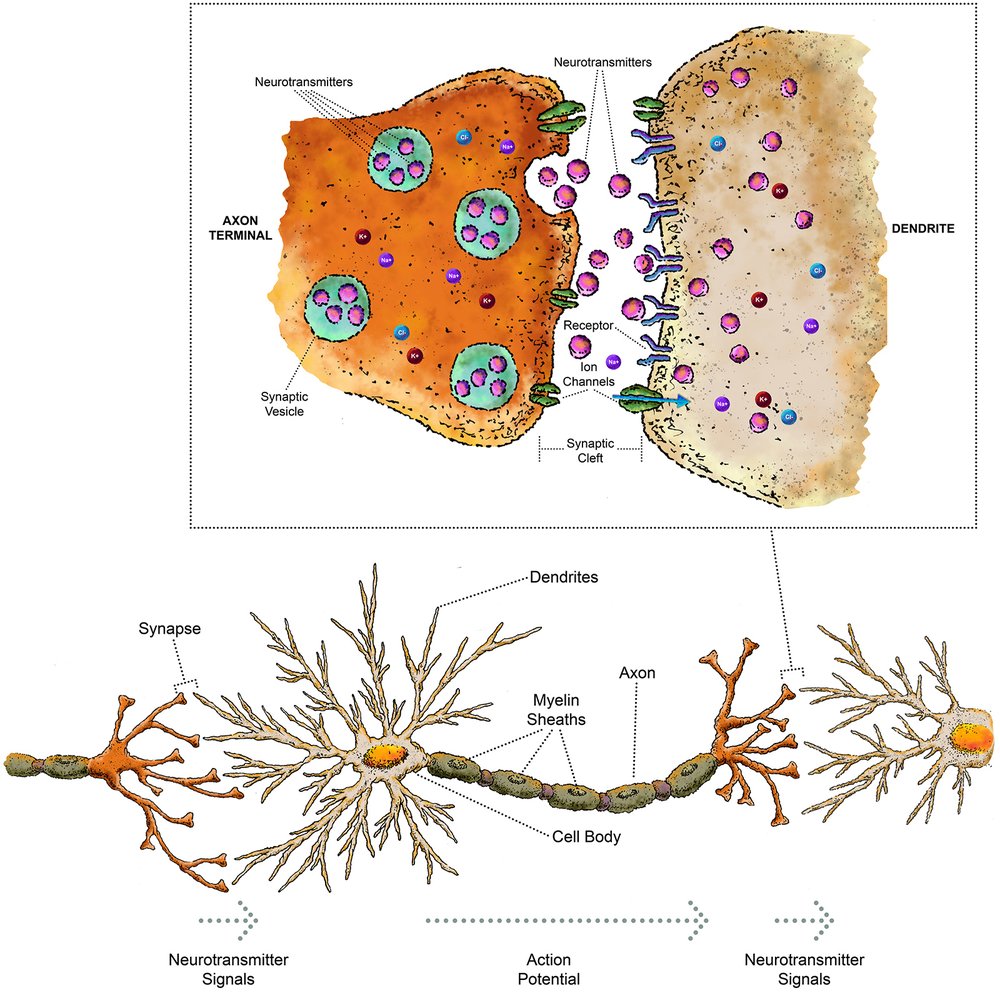
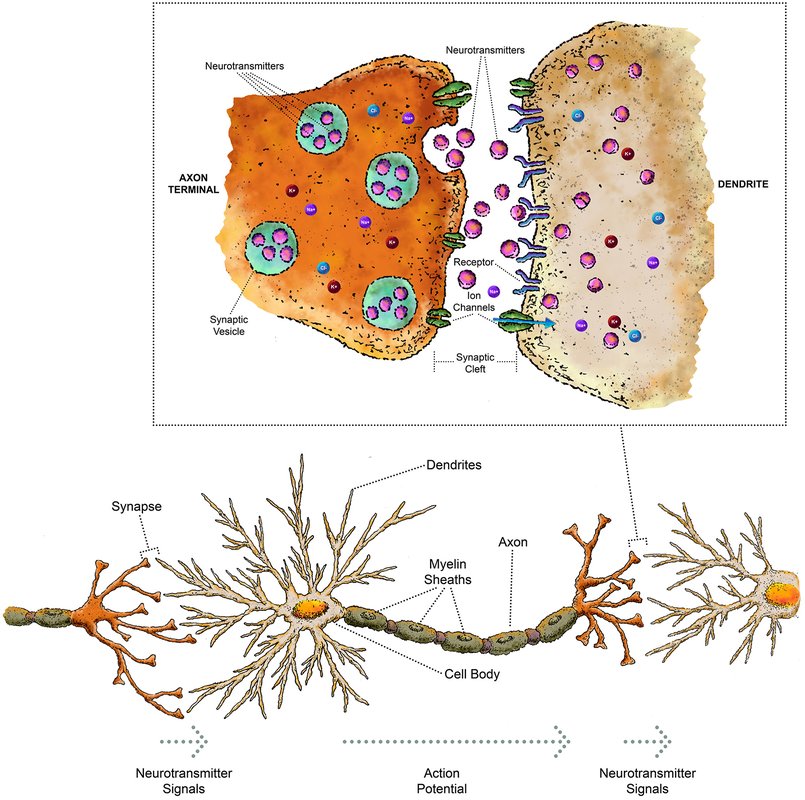
There is a chemical and an electrical component to neuronal signals. Dendrites receive signals in the form of chemical molecules called neurotransmitters. These neurotransmitters interact with receptors on the dendrites, which triggers the opening of channels that permit ions to pass in and out, changing the electric charge within the neuron (Figure 1 inset). If a certain threshold is met as a result of the change in charge, it sends the signal down along the axon. This is called an action potential. At the end of the axon, the action potential triggers the release of new neurotransmitters at the synapse to be picked up by the linked dendrites on nearby neurons.
The speed of the action potential is accelerated by myelin sheaths, insulating layers that wrap around the axon and let the signal "skip" from one section to the next. Specialized glial cells called astrocytes and microglia help with communication at the synapse, among their other functions.
A group of neurons working together to transmit a signal is called a neural circuit or a neural pathway.
Neuroplasticity
Our brains—and the neural pathways within them—change throughout our lives. They develop as we grow, and in adulthood they continue to adapt in response to new experiences. In many cases, the brain can even adapt to injuries.
This ability for our brains to change is called neuroplasticity. It is an important process that shapes learning, memory, and behavior.
Neurons can change at both the cellular and molecular levels. One neural pathway might become less stable and active, while another pathway might become more stable and active in response to something triggering it strongly or repeatedly (Figure 2). For example, you might walk into a dark room and be startled when your friends jump out of hiding and shout, "Surprise!" In the seconds after that event, a new pathway forms in the hippocampus creating a new memory.
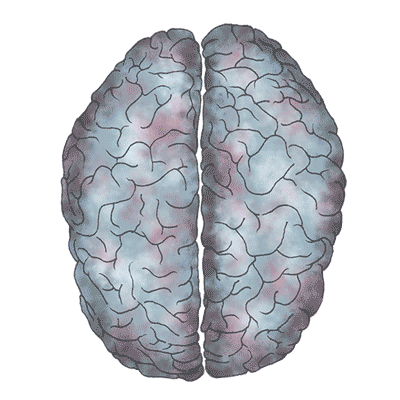
There are different mechanisms by which neuroplasticity can occur.
- Neurogenesis is the formation of new neurons. This occurs primarily during early development, but there is mounting evidence that some neurogenesis happens in adults.
- Synaptogenesis describes the formation of new synaptic contacts between neurons to build new neural pathways.
- Short-term enhancement, or "short-term facilitation," strengthens synaptic communication over the short term as a result of more frequent signals being sent from one neuron to another. This takes place on the order of tens of milliseconds to a few minutes. It is the result of more neurotransmitters being released from the axon at the synapse (compare figures 3a and 3b). This is called a "presynaptic" modification.
- Conversely, Short-term Depression (STD), a short-term decrease in signaling, results in fewer neurotransmitters being released from the presynaptic membrane (compare figures 3b and 3c).
- Long-term potentiation (LTP) creates long-lasting effects as more receptors are provided at the dendritic cell membrane, or the "postsynaptic" region (compare figures 4a and 4b). It also results in the postsynaptic cell structure being remodeled to increase the size and effectiveness of connections to dendrites and can cause sprouting of new synapses during development.
- Long-term Depression (LTD), a long-term decrease in signal, results in less effective signaling as a result of fewer receptors in the postsynaptic membrane (compare figures 4b and 4c).
Note that STD and LTD shouldn't be thought of as processes that disrupt learning and memory. They may be just as important as a means of getting rid of unimportant connections.
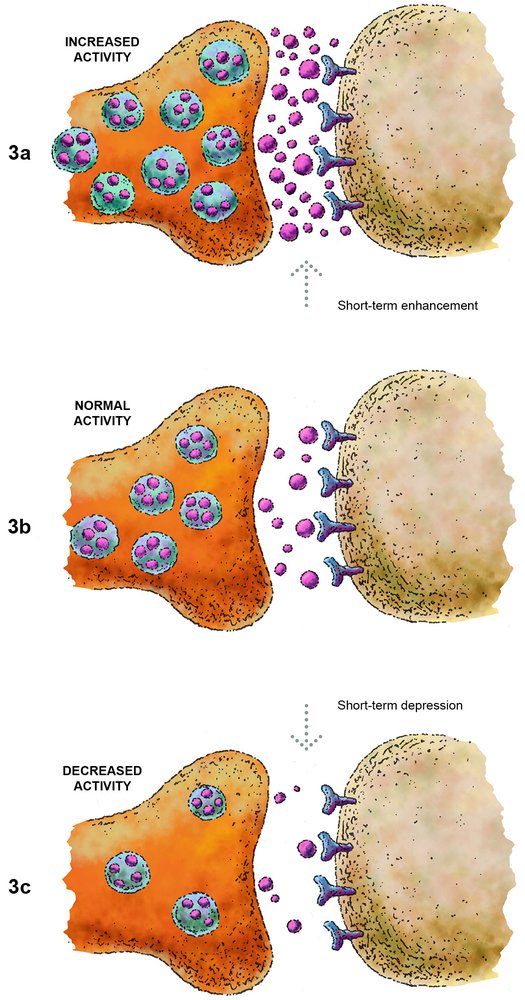
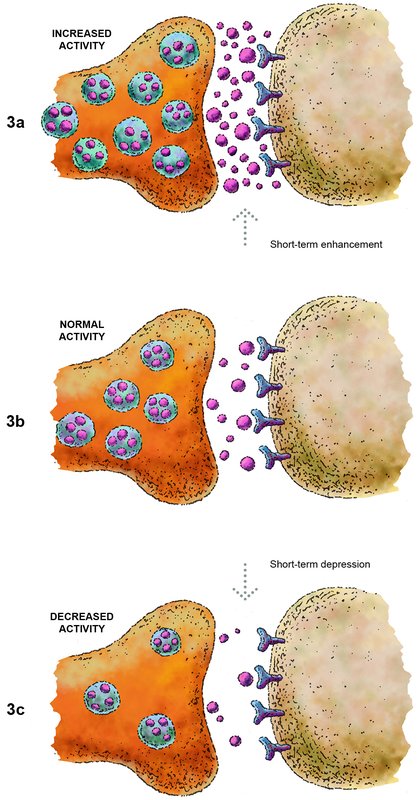
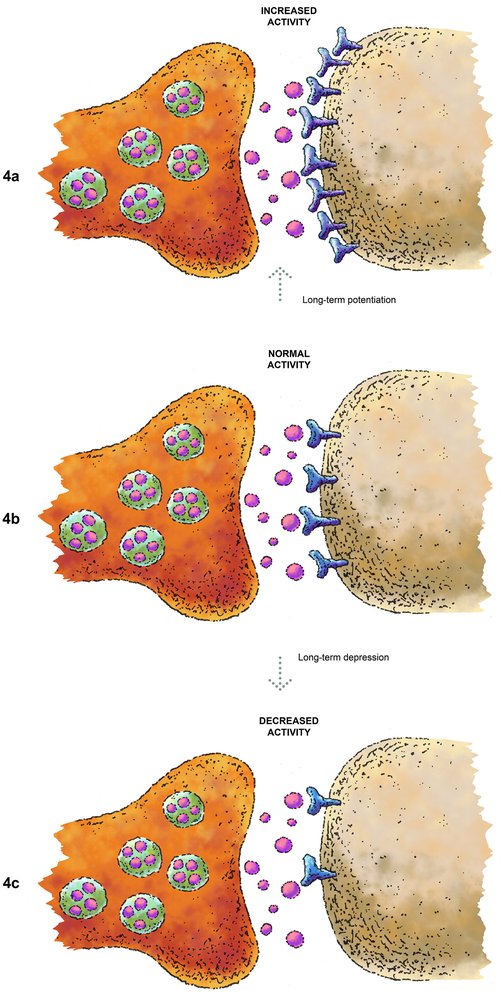
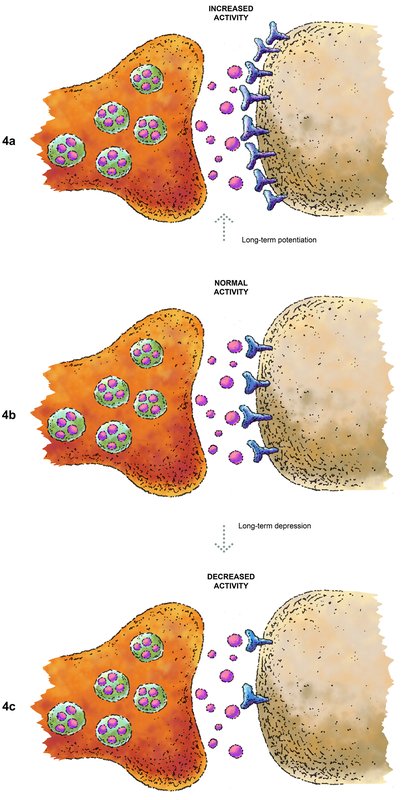
While neuroplasticity is thought to decline with age, there is evidence that it can be a lifelong process. Practicing new tasks in a way that is more demanding may lead to improved learning and memory.
Other occurrences of neuroplasticity take place primarily during childhood, but they may also be possible in adult brains. For example, synaptic pruning (Figure 5), which can begin near the time of birth and continue into our early 20s, involves the decay and removal of both axons and dendrites that are no longer needed. Irregularities during synaptic pruning may have a role in neurological disorders, including schizophrenia and autism spectrum disorder.
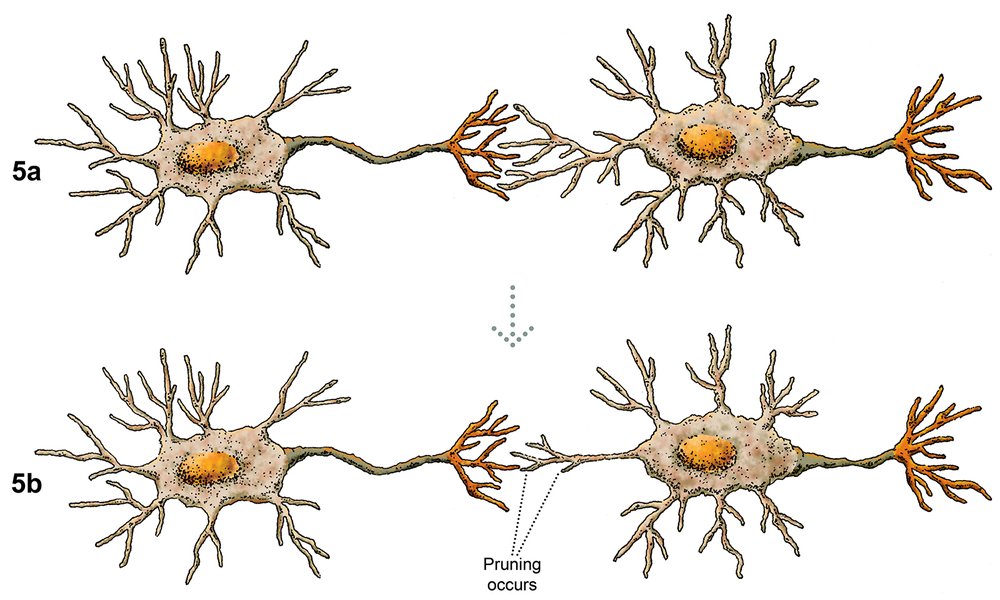
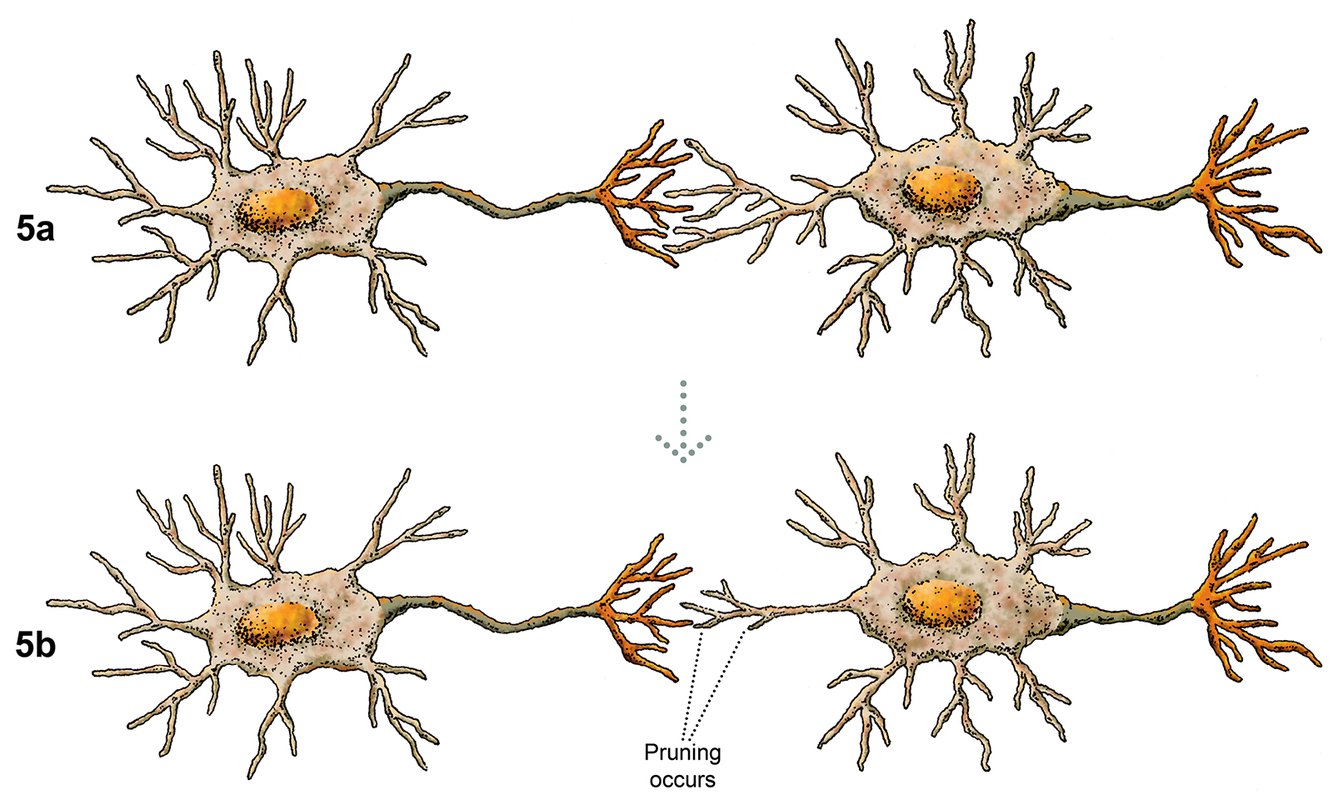
Neuroplasticity and brain injuries
Traumatic brain injuries can damage our neurons and disrupt neural pathways. In some cases, however, our brains take advantage of neuroplasticity to adapt after injury. This is referred to as functional neuroplasticity. Currently, four types of functional neuroplasticity have been identified.
Homologous area adaptation occurs when the function of a damaged part of the brain is taken over by a comparable part on the opposite half, or hemisphere. This process is more likely to occur among younger people, and the tasks originally associated with the region of the brain that must take on new functions may be compromised.
Compensatory masquerade occurs when the brain develops an alternative strategy for carrying out a task because the original strategy has been impaired. For example, if a person's ability to rely intuitively on their sense of direction, and distance is impaired, they could compensate by using landmarks to make their way to a destination.
If a region of the brain is deprived of signals coming in from our senses, cross-modal reassignment may allow new inputs of a different sensory type to access that brain area. A classic example of this occurs when a person who is blind receives touch sensory input in the part of the brain that usually receives visual input.
Map expansion describes what occurs when parts of the brain expand as a result of repeated practice. For example, intensive violin practice can cause expansion of the sensory areas of the brain that process finger sensations. Interestingly, this process has been associated with phantom limb syndrome, when a person experiences sensations in a limb they no longer have.
Researchers are studying whether functional neuroplasticity can be purposely induced as part of medical treatment. Cognitive training, exercise, brain stimulation, brain–machine interfaces, and therapeutics are all being explored with the hope of helping injured patients.