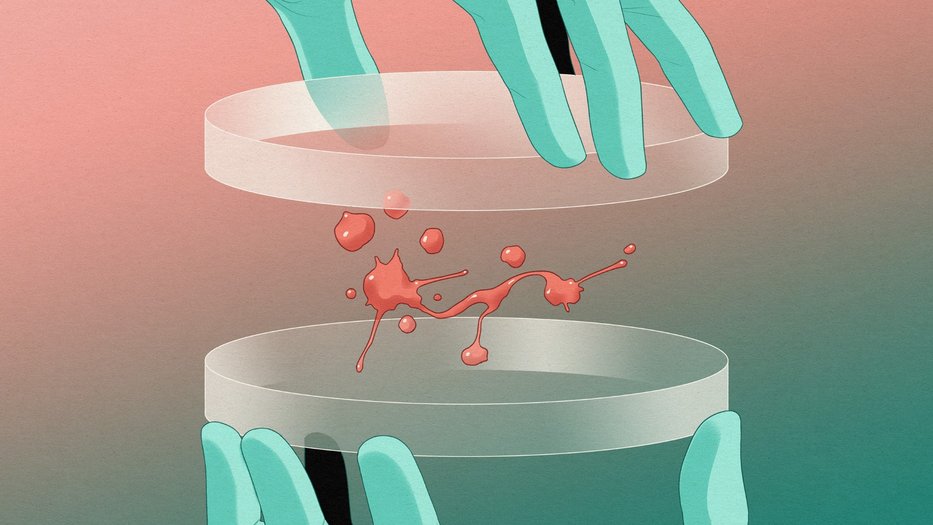
How Do Scientists Engineer Microbes, and What Are They Used For?
All microbes, or microorganisms, contain genetic material (DNA and/or RNA). In nature, this genetic material can change over time as a result of mutations and the transfer of genes between organisms, leading to microbial evolution. Scientists can manipulate and take advantage of these processes in the laboratory to produce specific and potentially useful characteristics in a microbe—such as the ability to repel crop pests—or to induce a microbe to produce substances, such as those needed for medicines.
Techniques
Humans have been using microbes to their advantage for thousands of years, first unknowingly cultivating them to make things like yogurt and beer.
Today, there are two main ways scientists in industry and in research laboratories intentionally modify microbes: directed evolution and genetic engineering.
Scientists can develop microbes with desired traits by coaxing them to mutate and evolve. How it works: In the lab, microbes are subjected to specific environmental conditions over the course of weeks to years. As the microbes replicate, some of the resulting microorganisms mutate, meaning their DNA slightly changes to adapt to the new environment. Scientists screen the new microbes to see which ones have developed the trait they are looking for and continue to breed those microbes in the lab. This technique is called directed evolution (also sometimes called adaptive laboratory evolution), and it has been around in some form for about 100 years. (In 2018, Caltech professor Frances Arnold received the Nobel Prize in Chemistry for her breakthrough work in directed evolution.)
Genetic engineering is a more targeted approach that reprograms a microbe's genetic instructions to give it a desired trait. Specific genes can be added, deleted, relocated, altered to respond to different environmental conditions; turned up (more strongly expressed); or turned down (more weakly expressed). The first genetically engineered microbes, using the bacteria E. coli, were created in 1973.
Scientists have used the technique of recombinant DNA technology to genetically engineer microorganisms since the 1970s. For example, the gene that produces insulin in humans can be inserted into a bacterium, coaxing that bacterium to produce insulin that can be purified and used as medicine. Recombinant DNA technology takes a cue from the way that bacteria fight bacteriophages (types of microbes that infect bacteria). When a bacteriophage inserts its DNA into a bacterium, the invaded bacterium uses something called a restriction enzyme (an enzyme is a type of protein) to cut up the DNA and fight the infection. In a similar way, scientists using recombinant DNA techniques can isolate pieces of DNA to make a microbe do something useful and insert them into the DNA of microbes.
Today, scientists can use even more sophisticated and precise techniques to engineer the genes of microbes, and engineer DNA that can be inserted into microbes. Currently, the most precise method is a gene editing tool called CRISPR-Cas9, whose technology has advanced over the last decade to allow scientists to add, delete, or modify a specific gene anywhere in a gene sequence. Previously, using recombinant DNA techniques, scientists had to find the right enzyme that would edit a gene sequence in the exact spot they were aiming for. CRISPR-Cas9 is able to edit anywhere in a gene sequence.
In gene editing, genes can be added to microbes from other microbes, plants, animals, and even humans. They can also be synthesized in a lab.
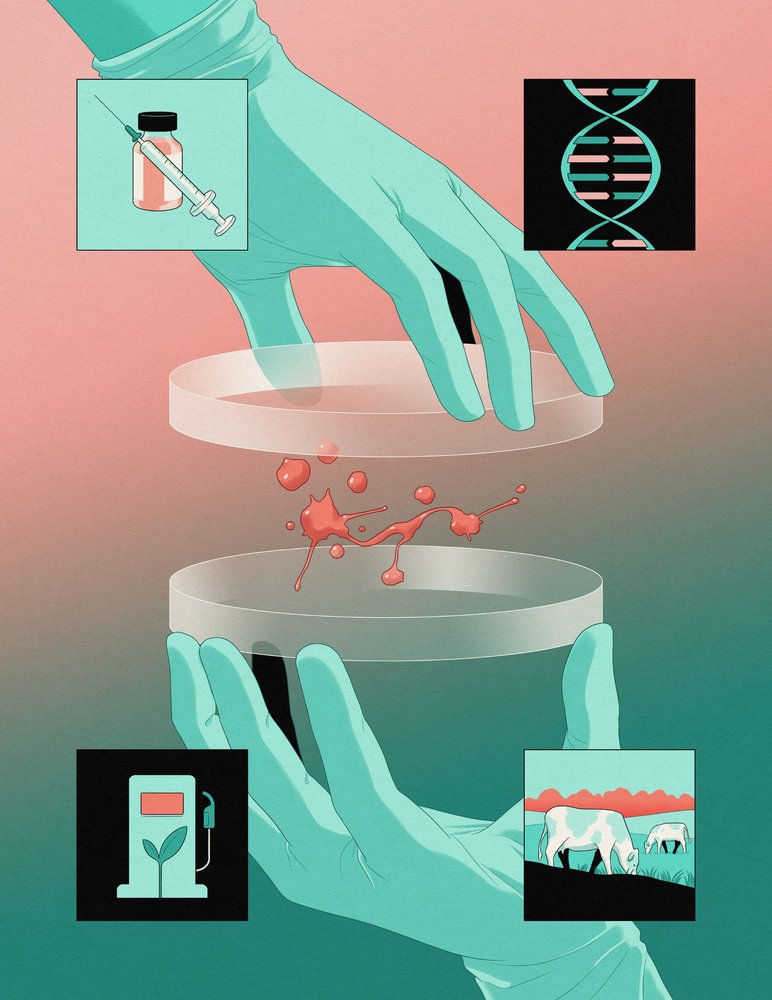
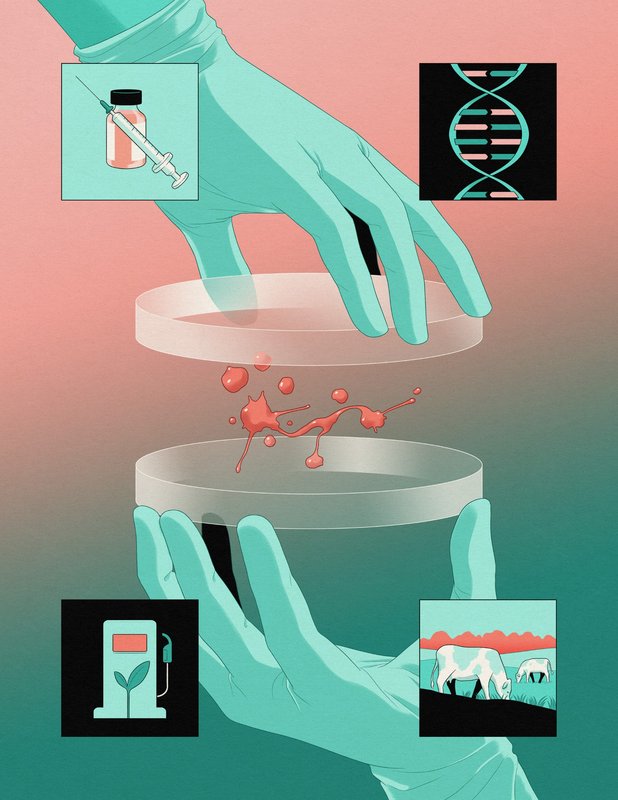
Applications
Genetically modified microorganisms have many current and potential uses across sustainability and energy; environmental cleanup and climate change mitigation; manufacturing; disease prevention, diagnosis, and treatment; and agriculture.
For example, most of the vanilla extract you buy at the grocery store is manufactured not from actual vanilla plants but produced synthetically due to the high cost and lack of availability of natural vanilla beans. As an alternative to the common practice of deriving the flavor from petrochemicals, it can be produced using yeast microbes. Fragrances, cosmetic products, food additives, and medicines are also manufactured this way.
Because the commercial use of engineered microbes has historically been focused on biomanufacturing, these microbes are not typically distributed in the wider environment. More recently, some engineered microbes have been approved by government regulatory agencies like the Food and Drug Administration and U.S. Department of Agriculture for use outside of the lab. For example, there is a genetically modified microbe marketed for humans to ingest to help reduce the effects of drinking alcohol, and others that are used to colonize the roots of crops so that farmers can use less fertilizer (which is expensive and creates greenhouse gases when manufactured).
Scientific advances over the past 20 or so years have unlocked the potential for engineered microbes to be deployed into the environment to autonomously perform useful tasks, such as detecting a toxic substance and degrading it. In the future, microbes could be used to alter the gut microbiome of livestock cows to reduce the amount of methane (a powerful greenhouse gas) that their burps release; to produce alternative fuels using organic waste (instead of the sugar and corn that are used today); to more cheaply and efficiently produce medicines for malaria and HIV; to decompose plastic waste; to make clothing that can give wearers data about their health; to create anti-corroding and self-repairing materials for infrastructure or vehicles; and the list goes on.
Safety Concerns
Many potential applications for engineered microbes would involve deploying them into an external environment, i.e., outside of the lab. This would carry potential risks alongside benefits.
How microbes spread and affect an ecosystem is an active area of scientific research. It is a complex area of study for a few reasons: First, microbes self-replicate, meaning they can grow in number quickly and are so small that they are extremely challenging to track and contain once they enter an ecosystem. Unlike nearly all plant and animal species, microbes frequently transfer their genes to other microbes around them (called horizontal gene transfer), so engineered genetic material in the new microbes can spread among existing microbe populations. Additionally, scientists have to consider a very long timeframe: Some microbes can form spores that can lay dormant in an environment for thousands of years and then resurface.
But the use of engineered microbes is not an entirely new concern: The risks associated with dispersing engineered microbes are similar to those for dispersing non-engineered microbes, such as when a species of microbe is brought from one country to another where it would not naturally travel.
Risks include a microbial species outcompeting a species native to an environment (upsetting the balance of an ecosystem) or causing harm to human health, for example, by getting into water supply or becoming infectious.
Further fundamental research is needed to better predict how microbes spread in and interact with an environment.
Currently, the U.S. regulatory framework implements strict safety requirements for the use of engineered microbes in the environment. As technological advances enable engineered microbes that can safely address new and useful applications, many parties (including the federal government itself) have advocated for revising existing regulations that could be too strict for a new generation of engineered microbial products. They argue that current standards would make it harder for society to take advantage of the benefits that engineered microbes have to offer, such as the ability to clean up otherwise intractable toxins from polluted land.